Reagents
Abbreviation | Full Name | Specifications |
---|---|---|
FITC-PAH | Poly(fluorescein isothiocyanate allylamine) Hydrochloride | MW = 15 kDa |
BPA | 4-Borono-L-phenylalanine | Purity ≥ 95.0% |
PSS | Poly(sodium 4-styrenesulfonate) | MW = 3.4 kDa |
FA | Folic Acid Dihydrate | Purity ≥ 95.0% |
DDIW | Double Deionized Water | R = 14.0 MΩ∙cm-1 |
NaCl | Sodium Chloride | Solid Pellets |
NaOH | Sodium Hydroxide | Solid Pellets |
GNP | Gold Nanoparticle Solution, Stabilized in Citrate Buffer | Diameter = 5 nm |
Procedures for Synthesis of Stock Solutions
This procedure is for the synthesis of the stock solutions used in the polyelectrolyte layering of the gold nanoparticles.
- All stocks are incubated at room temperature for at least 24 hours before use to ensure homogeneity and full boron conjugation
- Reagents measured using Thermo-Fisher Quintix mass balance, or Eppendorf Micropipettes
Stock Name | Reagents | Amount | Instructions |
---|---|---|---|
10 mL [3.5 Molal] B-FITC-PAHDDIW | FITC-PAH | 30 mg | Add reagents to beaker, then stir or vortex until homogeneous by inspection |
BPA | 0.5 mg | ||
DDIW | 10 mL | ||
4 mL [12 Molal] B-PSSDDIW | PSS | 40 mg | Add all reagents bar the BPA to a beaker, then bring up the pH of the solution to pH = 8 using the NaOH. Add the BPA and stir or vortex until homogeneous by inspection |
BPA | 8 mg | ||
DDIW | 4 mL | ||
NaOH | As Req. | ||
20 mL [2 Molal] FITC-PAHDDIW | FITC-PAH | 40 mg | Add reagents to beaker, then stir or vortex until homogeneous by inspection |
DDIW | 20 mL | ||
2 mL [10 Molal] PSSDDIW | PSS | 20 mg | Add reagents to beaker, then stir or vortex until homogeneous by inspection |
DDIW | 2 mL | ||
100 mL [0.5 M] NaClDDIW | NaCl | 2.922 mg | Grind the NaCl pellets using a mortar and pestle, then add reagents to beaker. Stir or vortex until homogeneous by inspection |
DDIW | 100 mL | ||
4 mL [10 Molal] PSSNaCl | PSS | 40 mg | Add reagents to beaker, then stir or vortex until homogeneous by inspection |
NaClDDIW | 4 mL | ||
55.8 mL [0.07 Molal] FANaCl | FA | 3.906 mg | Add reagents to beaker, then stir or vortex until homogeneous by inspection |
NaClDDIW | 55.8 mL |
Results
The relative reagent proportions for stock synthesis were referenced from Chanana et. al [1] and Mandal et. al [2]. When first attempting to make a B-PSSDDIW stock without pH = 8 solution, it was observed that a white particulate was still present in solution even after vigorous and prolonged vortexing (upwards of 10 minutes). After synthesizing PSSDDIW without the particulate observed, we deduced that the BPA was insoluble at the concentrations present, and so we raised the pH of the solution in order to solvate it. Indeed, it was confirmed that BPA has a low solubility of approximately 1.6 g/mL. All other solutions were successfully synthesized from referenced instruction.
Reagents
- Subscript denotes solvent type
Abbreviation | Full Name | Specifications |
---|---|---|
B-FITC-PAHDDIW | BPA conjugated FITC-PAH Solution | Conc. = 3.5 Molal |
B-PSSDDIW | BPA conjugated PSS Solution | Conc. = 12 Molal |
FITC-PAHDDIW | FITC-PAH Solution | Conc. = 2 Molal |
PSSDDIW | PSS Solution | Conc. = 10 Molal |
PSSNaCl | PSS Salt Solution | Conc. = 10 Molal |
DDIW | Double Deionized Water | R = 14.0 MΩ∙cm-1 |
NaClDDIW | Sodium Chloride Solution | Conc. = 0.5 M |
Procedures for Boron-Polyelectrolyte GNP Layering
This procedure layers our gold nanoparticle in alternatingly charged polyelectrolyte layers that are conjugated with boron. A total of 6 layers are electrostatically bound to the GNP in succession. The first four layers are boron-conjugated polyelectrolytes: 4-Borono-L-Phenylalanine:Poly(fluorescein isothiocyanate allylamine hydrochloride) and 4-Borono-L-Phenylalanine:Poly(sodium 4-styrenesulfonate). The fifth layer is a non-boron conjugated FITC-PAH solution in double deionized water. The sixth layer is a non-boron conjugated PSS solution in 0.5 M sodium chloride.
- This procedure is carried out over three consecutive days
- 5 Samples (G1 - G5) were run simultaneously throughout the procedure
- Eppendorf Micropipettes were used for all volume drop or transfer
- An Eppendorf 5424 Micro-centrifuge was used for all centrifugation
- VWR polypropylene micro-centrifuge tubes were used for the procedure
- A Malvern Zetasizer Nano Z was used to conduct DLS and Zeta-Potential measurements
Day 1
Time Since Start of Work (hrs.) | Instruction |
---|---|
00:00 | Add 1 mL of GNP stock solution drop-wise to 0.5 mL of B-FITC-PAHDDIW in a 1.5 mL micro-centrifuge tube. Vortex the sample for approximately 30 seconds |
00:10 | Label the micro-centrifuge tube with [sample #] and I (for 1st layer), then incubate for 20 minutes in a dark environment |
00:30 | Create one 1.5 mL water counterbalance, then centrifuge samples at 18,000 g (RCF) for 60 minutes |
01:30 | Decant the supernatant, then resuspend the pellet up to 1.5 mL with DDIW |
01:50 | Leave to resuspend for 120 minutes in a dark environment |
03:50 | Create one 1.5 mL water counterbalance, then centrifuge samples at 18,000 g (RCF) for 60 minutes |
04:50 | Decant the supernatant, then resuspend the pellet up to 1.5 mL with DDIW |
06:50 | Leave to resuspend for 120 minutes in a dark environment |
08:50 | Conduct Dynamic Light Scattering to determine hydrodynamic radius, and Zeta-Potential to determine surface charge |
09:50 | Add the sample drop-wise to 0.2 mL of B-PSSDDIW in a new 1.5 mL micro-centrifuge tube. Vortex the sample for approximately 30 seconds |
10:00 | Label the micro-centrifuge tube with [sample #] and II (for 2nd layer), then incubate for 20 minutes in a dark environment |
10:20 | Create one 1.5 mL water counterbalance, then centrifuge samples at 18,000 g (RCF) for 60 minutes |
11:20 | Decant the supernatant, then resuspend the pellet up to 1.5 mL with DDIW |
11:40 | Leave to resuspend for 120 minutes in a dark environment |
13:40 | Create one 1.5 mL water counterbalance, then centrifuge samples at 18,000 g (RCF) for 60 minutes |
14:40 | Decant the supernatant, then resuspend the pellet up to 1.5 mL with DDIW |
15:00 | Leave to resuspend for 120 minutes in a dark environment |
17:00 | Conduct Dynamic Light Scattering to determine hydrodynamic radius, and Zeta-Potential to determine surface charge |
18:00 | Store samples in a dark environment at room temperature |
Day 2
Time Since Start of Work (hrs.) | Instruction |
---|---|
00:00 | Add samples drop-wise to 0.5 mL of B-FITC-PAHDDIW in a 1.5 mL micro-centrifuge tube. Vortex the sample for approximately 30 seconds |
00:10 | Label the micro-centrifuge tube with [sample #] and III (for 3rd layer), then incubate for 20 minutes in a dark environment |
00:30 | Create one 1.5 mL water counterbalance, then centrifuge samples at 18,000 g (RCF) for 60 minutes |
01:30 | Decant the supernatant, then resuspend the pellet up to 1.5 mL with DDIW |
01:50 | Leave to resuspend for 120 minutes in a dark environment |
03:50 | Create one 1.5 mL water counterbalance, then centrifuge samples at 18,000 g (RCF) for 60 minutes |
04:50 | Decant the supernatant, then resuspend the pellet up to 1.5 mL with DDIW |
06:50 | Leave to resuspend for 120 minutes in a dark environment |
08:50 | Conduct Dynamic Light Scattering to determine hydrodynamic radius, and Zeta-Potential to determine surface charge |
09:50 | Add the sample drop-wise to 0.2 mL of B-PSSDDIW in a new 1.5 mL micro-centrifuge tube. Vortex the sample for approximately 30 seconds |
10:00 | Label the micro-centrifuge tube with [sample #] and IV (for 4th layer), then incubate for 20 minutes in a dark environment |
10:20 | Create one 1.5 mL water counterbalance, then centrifuge samples at 18,000 g (RCF) for 60 minutes |
11:20 | Decant the supernatant, then resuspend the pellet up to 1.5 mL with DDIW |
11:40 | Leave to resuspend for 120 minutes in a dark environment |
13:40 | Create one 1.5 mL water counterbalance, then centrifuge samples at 18,000 g (RCF) for 60 minutes |
14:40 | Decant the supernatant, then resuspend the pellet up to 1.5 mL with DDIW |
15:00 | Leave to resuspend for 120 minutes in a dark environment |
17:00 | Conduct Dynamic Light Scattering to determine hydrodynamic radius, and Zeta-Potential to determine surface charge |
18:00 | Store samples in a dark environment at room temperature |
Day 3
Time Since Start of Work (hrs.) | Instruction |
---|---|
00:00 | Add samples drop-wise to 0.5 mL of FITC-PAHDDIW in a 1.5 mL micro-centrifuge tube. Vortex the sample for approximately 30 seconds |
00:10 | Label the micro-centrifuge tube with [sample #] and V (for 5th layer), then incubate for 20 minutes in a dark environment |
00:30 | Create one 1.5 mL water counterbalance, then centrifuge samples at 18,000 g (RCF) for 60 minutes |
01:30 | Decant the supernatant, then resuspend the pellet up to 1.5 mL with DDIW |
01:50 | Leave to resuspend for 120 minutes in a dark environment |
03:50 | Create one 1.5 mL water counterbalance, then centrifuge samples at 18,000 g (RCF) for 60 minutes |
04:50 | Decant the supernatant, then resuspend the pellet up to 1.5 mL with DDIW |
06:50 | Leave to resuspend for 120 minutes in a dark environment |
08:50 | Conduct Dynamic Light Scattering to determine hydrodynamic radius, and Zeta-Potential to determine surface charge |
09:50 | Add the sample drop-wise to 0.2 mL of PSSNaCl in a new 1.5 mL micro-centrifuge tube. Vortex the sample for approximately 30 seconds |
10:00 | Label the micro-centrifuge tube with [sample #] and IV (for 4th layer), then incubate for 20 minutes in a dark environment |
10:20 | Create one 1.5 mL water counterbalance, then centrifuge samples at 18,000 g (RCF) for 60 minutes |
11:20 | Decant the supernatant, then resuspend the pellet up to 1.5 mL with NaClDDIW |
11:40 | Leave to resuspend for 120 minutes in a dark environment |
13:40 | Create one 1.5 mL water counterbalance, then centrifuge samples at 18,000 g (RCF) for 60 minutes |
14:40 | Decant the supernatant, then resuspend the pellet up to 1.5 mL with NaClDDIW |
15:00 | Leave to resuspend for 120 minutes in a dark environment |
17:00 | Conduct Dynamic Light Scattering to determine hydrodynamic radius, and Zeta-Potential to determine surface charge |
18:00 | Store samples in a dark environment at room temperature |
Results
Initial centrifugation did not produce stable pellets, and so centrifugation time was increased with respect to the referenced procedure by Chanana et al. [1]. Times for incubation were also modified, as we predicted that changing incubation times would not have significant impact on results.
The GNP solution and subsequently layered samples are always added drop-wise to the next layer of polyelectrolytes in order to avoid colloidal destabilization. Incubation in dark environments is also done for the same purpose.
Our first attempts at GNP polyelectrolyte layering was intended to be split into 9 hour work days with 1 layer per day. However, initial results of our first attempts at GNP layering returned expected hydrodynamic radii and low PDI on the first DLS run, but extremely large hydrodynamic radii on the second DLS run along with a high PDI. This indicated that aggregation had most likely occurred during the incubation of the singly layered B-FITC-PAHDDIW GNP. After deliberation with team members, it was concluded that the most likely cause of aggregation was a competitive removal of the stabilizing citrate dianion shell by the polycationic electrolyte. Normally, GNPs are prone to aggregation at even slight temperature, light, or physical disturbance due to their low, slightly negative surface potential. Therefore, citrate buffer is used to stabilize the GNPs in a dianion shell, which effectively increases the surface charge, creating a stabilizing electrostatic repulsion between particles. However, after removal of this citrate layer by the polycationic electrolyte, the electrostatic repulsion was insufficient for keeping the particles stabilized, and aggregation occurred while the particles were incubated overnight. The first Zeta-Potential measurement of the first polycationic layer agrees with this hypothesis, indicating a positive, but low surface charge (approximately +5 mV) on the singly layered particles. The second Zeta-Potential measurement, however, returned sufficiently high values (approximately -50 mV). It was decided then that two layers would have to be done per day in order to end the day on a polyanionic electrolyte layer so that the particles would not aggregate during incubation overnight.
Final results show that our nanoparticles are approximately 100 nm in diameter with a slightly negative surface charge. This result was expected, as Mandal et al. [2] also observed a particle collapse and predicted a reduced stability due to sodium chloride being used as a solvent for the outer layer (the ideal theoretical diameter is 125 nm - 5 nm for the core, and 20 nm of diameter per layer added). Our results convey maximum colloidal stability to be reached at the fourth polyelectrolyte layer. However, we decided to use 6 layers, as the outer layer solvent of sodium chloride is important for biocompatibility. Transmission Electron Microscope (TEM) characterization confirmed that our layered nanoparticles were approximately 100 nm in diameter, and that aggregation had occurred to a moderate level due to the low surface potential. Black dots of high electron density were observed in the TEM images, which represent the BPA conjugated into the polyelectrolyte layers.
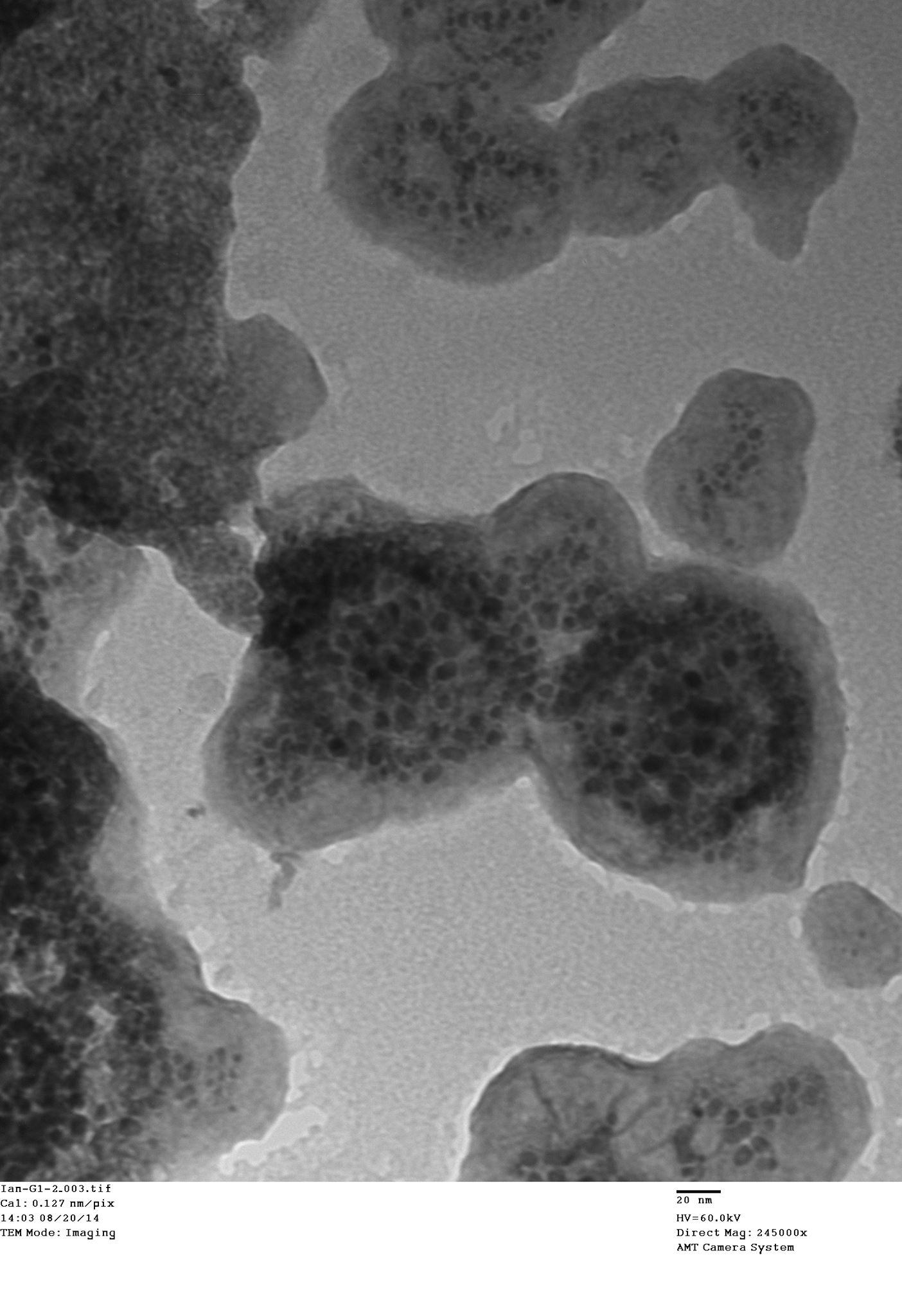
Reagents
- Subscript denotes solvent type
Abbreviation | Full Name | Specifications |
---|---|---|
FANaCl | Folic Acid Salt Solution | Conc. = 0.07 Molal |
HCl | Hydrochloric Acid | %m/m = 37% |
DDIW | Double Deionized Water | R = 14.0 MΩ∙cm-1 |
MES | 2-(N-morpholino) Ethanesulfonic Acid | Purity ≥ 99.5% |
EDC | N-(3-Dimethylaminopropyl)-N’-ethylcarbodiimide Hydrochloride | Purity ≥ 98% |
Sulfo-NHS | N-hydroxysulfosuccinimide Sodium Salt | Purity ≥ 98% |
β-M | 2-Mercaptoethanol | Purity ≥ 99.0% |
BDNA | Biotinylated DNA | From Bio Basic |
Walker | DNA Tensegrity Walkers | From Walker Synthesis |
Tris | Trizma Hydrochloride | Purity ≥ 99.0% |
LGNP | Layered Gold Nanoparticles | From Gold Nanoparticle Polyelectrolyte Layering |
Procedure for Folic Acid Functionalization
Time Since Start of Work (hrs.) | Instruction |
---|---|
00:00 | Weigh out micro-centrifuge tubes and record masses |
00:20 | Dilute HCl to pH = 1.35 (0.045 M) using DDIW |
00:30 | Add 1 mL of a 50% v/v ratio of FANaCl:HCl to each micro-centrifuge tube |
00:35 | Add 50 μL of the Layered GNP sample drop-wise to the micro-centrifuge tube until a total volume of 1.5 mL has been reached (0.5 mL of LGNP) |
00:45 | Create one 1.5 mL water counterbalance, then centrifuge samples at 5,000 g (RCF) for 90 minutes |
01:00 | Prepared MES buffer with 0.0389 g MES in 5 mL of DDIW |
01:10 | Weigh out two 0.4 mg of EDC and two 1.1 mg of Sulfo-NHS |
02:15 | Decant 1.4 mL of supernatant, leaving 0.1 mL of a yellow pellet in the micro-centrifuge tube |
02:45 | Weigh out all micro-centrifuge tubes with pellets |
03:15 | Add 0.9 mL of MES to the pellets, checking that the pH = 6.0 with pH paper |
03:30 | Transfer LGNP samples to two new micro-centrifuge tubes each with 0.4 mg of EDC and 1.1 mg of Sulfo-NHS in them |
03:45 | Incubate the LGNP-EDC-Sulfo-NHS samples in a dark environment for 15 minutes |
04:00 | Add 1.4 μL of β-M to each of the samples to quench the reaction |
04:05 | Add 10 μL of BDNA to the Walker sample, then incubate in a dark environment for 60 minutes |
05:05 | Add the BDNA-Walker complex to the FA functionalized LGNP samples |
05:20 | Incubate samples in a dark environment for 120 minutes |
07:20 | Add 0.00242 g of Tris to the samples |
Results
The conjugation of folic acid to the LGNPs was done by protonating the folic acid primary amine group (pKa = 2.35) to make a positively charged, resonance stabilized analogue of folic acid. This electrostatically binds with the anionic sulfate groups of the PSS layer of the LGNP. This leaves the nanoparticles with carboxyl groups on the surface to react for the coupling reaction. The successful reaction was visible, since the folic acid solution was yellow. Upon the reaction of the LGNPs with the folic acid, the resulting pellet after centrifugation was yellow, while the rest of the solution was clear. This indicated that the folic acid was tethered to the LGNPs.
The biotinylated DNA is conjugated onto the DNA via direct reaction. In our case, the cassette and tile system is not required because we are only adding one functional attachment to the walker. In the case that more attachments are required, the walkers can be walked across the tile using a preset thermocycler program.
This coupling method was modified from a procedure by Gabarek and Gergely [3] (used from Fischer Scientific Product Instructions). It links a carboxy-terminated molecule with an amine-terminated molecule with a zero-length crosslink. The procedure was used to link the carboxylic group on folic acid to the amine group(s) on the walkers. This procedure was not verified through characterization methods due to constraints in time and resources. However, the AFM results of the procedure will be presented at Biomod 2014.